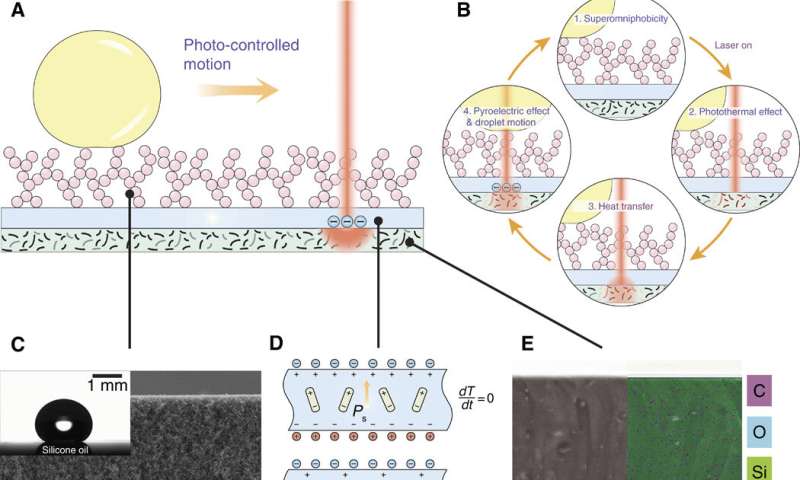
Precisely manipulating various liquids is essential in many fields and unlike solid objects, fluids are intrinsically divisible. Fluids are also sticky with appropriate functions for lossless manipulation to prevent loss and contamination. In a new report now published on Science Advances, Wei Li and colleagues in mechanical engineering and research and innovation in China presented photopyroelectric microfluidics to meet such diverse requirements. The fluidic platform facilitated the development of a unique wavy dielectrophoretic force field from a single beam of light to remarkably perform the desired loss-free manipulation of droplets and function as a “magic” wetting-proof surface. The liquid platform could navigate, fuse, pinch and cleave fluids on demand to establish cargo carriers with droplet wheels and has potential to upgrade the maximum concentration of deliverables such as protein by 4000-fold.
Existing methods to merge fluids
The surface manipulation of buffers and organic solvents is fundamental for many biological applications and chemical functions that are critical for a variety of thermal, optical and medical applications. To accomplish this, scientists must design a platform to enable locally addressable fluids for navigation with a low loss rate to partition and merge in a readily controlled process. Light can outperform other stimuli due to its contactless nature, high precision, and mature ray controllability relative to geometric optics, for example, to form optical tweezers that trap and dislodge micro-objects. Several approaches have therefore explored the potential to photo-manipulate liquids by leveraging the energy conversion of photoelectric, photothermal, photochemical and photomechanical properties to precisely navigate and merge fluids. Nevertheless, these techniques cannot split and manipulate fluids in a loss-free manner. Therefore, in this work, Li et al. presented an unprecedented approach.
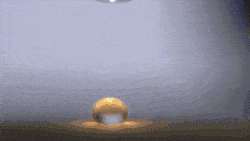
The new approach
The team simply stacked three homogenous layers, including a photothermal film using a graphene-doped polymer, pyroelectric crystal using a lithium niobate wafer, and a superomniphobic surface using a silica nanosphere. The three layers functioned in concert for loss-free applications of even, ultra-low surface tension fluids in the presence of a single beam of light.
They composed the photothermal film with a graphene monolayer composite to sense the light stimuli and sense the responses generated by uneven thermogenesis. The pyroelectric crystal converted heat into extra electric charges to form a wavy dielectrophoretic force profile that could trap, dispense and split the fluids. They employed the technique to perform four fundamental functions including movement, merging, dispensing and splitting of various liquids under well-controlled, loss-free conditions without complicated electrodes and high-voltage circuits. The approach will have significant impact across multidisciplinary fields.
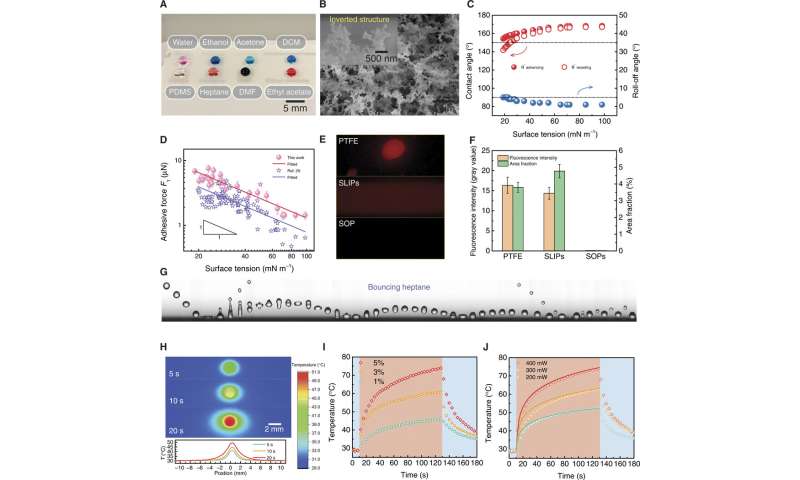
Designing photopyroelectric microfluidics
Li and team used the three layers of closely sandwiched materials (the pyroelectric crystal, superomniphobic thin film and photothermal thin film) to form the platform. The top superomniphobic layer contained nanoscale fractal networks made by sintering hollow silica spheres covered with fluorinated surfactants to achieve super-repellence. In the bottom layer, they formed a uniform composite film by homogenizing graphene nanoplatelets with polydimethylsiloxane (PDMS) and cured the polymer. When a beam of near-infrared (NIR) light irradiated the surface, the translucent superomniphobic surface and pyroelectric wafer became a transparent window allowing the NIR to readily reach the underlying composite polymer film. This led to a partially uneven, localized temperature rise, giving form to extra surface free charges, allowing droplets on the superomniphobic surface to be driven forward to the irradiated spot via a dielectric force. The scientists applied the technique to a variety of liquids including organic solvents such as silicone oil, alkanes and alcohols. The platform provided a channel-free, open-space fluidic processor without the hassle of electrodes or micropatterning required for currently existing microfluidic counterparts.
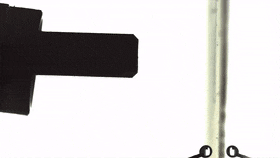
Loss-free fluid interfacing, light sensitive sensing, and droplet dynamics
The superomniphobic surface was chemically resistant to corrosive acids and bases, allowing a stable cassie state to remain on the surface for chemical fluidic processing. The scientists confirmed loss-free fluid interfacing via fluorescence imaging of the omniphobic surface and compared the results with controls to show near loss-free contact with fluids on the material of interest. Li et al. thereafter noted the light-sensing capacity of the system to show the conversion of irradiated light into a sharply bulged temperature profile in the system. They then investigated the motion of a 5 microliter (µl) droplet of water placed 13 mm away from the light spot center. When they turned the laser on, the droplet was attracted to the illumination in an oscillating mode, where it initially accelerated toward the laser, then rapidly braked and reversed direction on reaching the light spot’s edge. To understand the underlying physics of droplet dynamics, the team developed a numerical simulation and varied the liquid types for the calculations to show that higher the relative permittivity and surface tension, the easier for liquid motion.
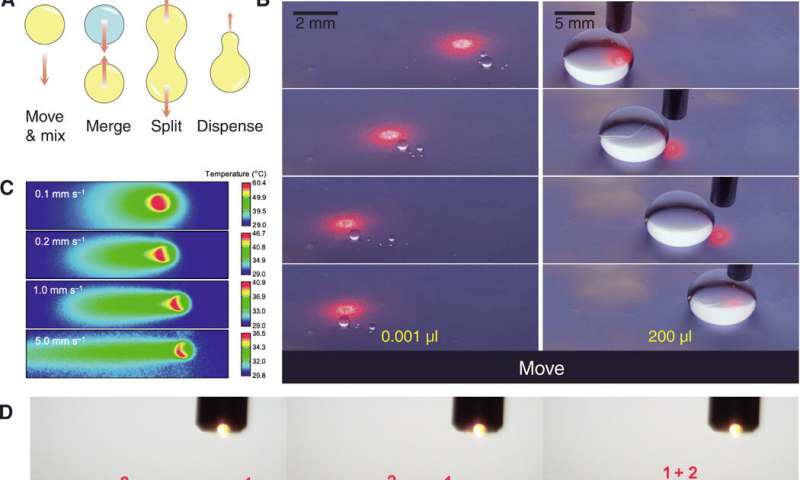
Fluidic functionality, versatility, and biocompatibility
The team performed a variety of fluidic functions using a single beam of laser light, where the wavy dielectrophoretic force profile could unexpectedly trap and move droplets with a volume as low as 0.001 µL. The team also handled a 200 µL puddle without loss on the platform, suited for miniaturization of biomedical systems. However, the technique had its limits with a maximum laser-moving velocity beyond which the droplet could not keep up with laser movement. Additionally, Li et al. facilitated a strong navigating force for droplets to defy gravity and ascend uphill by placing the platform vertically, allowing the superior technique to precisely manipulate various liquids at the micro-/nanoliter scale, which is of fundamental importance across multiple fields. Using the method, the team observed the loss-free detection of amino acids such as glycine and low-surface tension liquids such as ethanol. The method has great potential in analytical chemistry, medical diagnosis, and biomedicine.
In this way, Wei Li and colleagues developed a unique wavy dielectrophoretic force field in response to light stimuli with a three-layered surface for well-controlled, loss-free liquid motion, merging, dispensing and splitting functionalities. They readily modified the force by superimposing multiple light irradiations for richer fluidic functionality and droplet patterning applications. The method will facilitate fluid maneuver on demand for applications in biochemical and fluidic processing reactions, fluidic engineering and manufacturing for precision patterning and for droplet multi-compartmentalization.
Wei Li et al. Photopyroelectric microfluidics, Science Advances (2020). DOI: 10.1126/sciadv.abc1693
Qiangqiang Sun et al. Surface charge printing for programmed droplet transport, Nature Materials (2019). DOI: 10.1038/s41563-019-0440-2
Youmin Hou et al. Recurrent Filmwise and Dropwise Condensation on a Beetle Mimetic Surface, ACS Nano (2014). DOI: 10.1021/nn505716b
Science Advances
,
Nature Materials
,
ACS Nano